Mom Was Wrong
When Cheaters WinMyxobacteria are characterised by a complex life cycle. Bacteria Break Rules, Act Selfish, and ThriveMom was wrong. Sometimes, cheaters do win - even in the incredibly small, and surprisingly complex, world of bacteria. Gregory Velicer, a professor at Michigan State University’s Center for Microbial Ecology, has found that some bacteria not only survive when they break the rules, they thrive. Velicer’s research is part of the pioneering work in biologist Richard E. Lenski’s lab on the East Lansing campus. While others concentrate on higher forms of life in their quest for a better understanding of evolution, Lenski and his researchers look at some of the most primitive organisms on the planet. Evolution While You WaitSince 1988, Lenski has been studying 12 populations of the bacterium E coli. During that time, the bacteria have gone through more than 24,000 generations, something that would take humans 500,000 years to do. The advantage of studying bacteria is it lets the scientists observe evolution - and even prod it along - over many generations. They can literally watch evolution unfold before their eyes. By subjecting E coli to a feast and famine regimen, for example, the scientists found that the bacteria learned to thrive under stress. In all 12 populations, the size of the cells doubled. The Social Life of Bacteria
The real drama takes place inside the fruiting body. Some of the rod-shaped bacteria are transformed into a reproductive organism called a spore. The other members of the strain are supposed to make the final sacrifice for the good of the community. They are supposed to die, literally spilling their guts and releasing chemicals that will allow other cells to become spores. That’s the only way the community can make it through the famine. Bacteria Who Just Say NoBut guess what? Some of the bacteria cheat, refusing to become spores or die off for the good of the community. Velicer says he had expected to find some cheating, but "what surprised us was how easily we found it, and how frequent it appeared to be." Velicer used the example of a wolf pack. Some wolves, he says, might invite themselves to dinner even if they didn’t participate in the hunt. But "it requires more than simply freeloading" to qualify as a cheater, he adds. Instead of just getting a nibble or two, the cheating wolf gets more food than the wolves that killed the prey. Likewise, the cheaters in Velicer’s bacteria also got more than their share. Breaking the Rules and WinningVelicer observed the bacteria for 150 days, during which it went through about 1,000 generations. He found that the cheating bacteria couldn’t form spores on their own, making them seem developmentally disabled. He extracted the cheaters and put them aside for further experimentation. When he mixed the cheaters in with a wild strain of Myxococcus, the wild ones sent out some kind of chemical signal and the former cheaters began forming spores with gusto. In fact, they were better at it than the wild types. In a word, Velicer says, they thrived, even though they had cheated to get there. But there is a limit. If he mixed in too many cheaters, there weren’t enough wild bacteria to provide the chemical signals to trigger the cheaters to form spores, many of the cheaters died off. So some cheaters can actually enhance the strength of the community, but if there are too many, some of them will die, keeping the strain in balance. Finding Cheaters in NatureVelicer, who published his research in a recent issue of the journal Nature, is now moving into the next phase of his research. He plans to comb through the mud, and whatever else it takes, to capture enough wild bacteria to see if the same thing is happening in nature. "These cheaters were so easy to find in the lab that we strongly suspect there are frequent cheaters in nature," he says. To some, the social habits of bacteria may not seem all that important, but bacteria are in fact the dominant living creatures on Earth. Some cause disease, though most are harmless, and many contribute in various ways to the health and vitality of higher life forms. And they have been around for a long, long time. Some experts believe bacteria formed at least 3 billion years ago, when Earth itself was fairly young. By 1.5 billion years ago, they were all over the place. Without them, there would not have been enough oxygen in the atmosphere for higher life forms to survive. And just imagine, cheaters played a role in it all. Source: Lee Dye’s column which appears Wednesdays on ABCNEWS.com 19 April 2000. A former science writer for The Los Angeles Times, Lee now lives in Juneau, Alaska. Bacteria Uses Sonar-like Strategy to Probe Environmentby Ker Than An infection-causing bacteria uses a unique sonar-like strategy to scan its environment, similar to that used by bats when hunting in the dark, according to a new study. The research solves a 70-year-old medical mystery and could lead to the development of new drugs that target bacterial infections without spurring antibiotic resistance. Bacteria are microscopic single-celled organisms, some of which are responsible for human infections and disease. The bacteria Enterococcus faecalis has interested researchers ever since 1934, when it was discovered that it could produce a substance called cytolysin that can poison or kill a broad range of organisms. Scientist have since learned that the toxin is composed of two protein subunits, one small and one large, that are constantly being produced by the bacteria at low levels. It was observed that E faecalis was somehow able to sense the presence of nearby target cells and ramp up toxin production in response, but until now, scientists were in the dark as to how this was accomplished. The research team, led by Michael Gilmore, director of the Schepens Eye Research Institute at Harvard Medical School, solved the mystery when they discovered that the cytolysin subunits also act as a two-part environmental probe. Like armed sentries sent out in pairs, they scout the terrain, and when they come across an enemy, one will attack, while the smaller of the two rushes back with a message for reinforcements. Here's how it works: If a target cell is present, the larger subunit will bind to it, leaving its smaller half unattended and free to report back to the bacterium. The return signal kicks toxin production from its normally low levels into high gear. In the absence of a target cell, the large subunits latch onto the smaller ones and keep them below the level needed to trigger an increase in toxin production. Although the bacteria do not emit high pitched sounds the way bats do when they navigate in the dark, the basic principle is the same. "You send out a signal, and you look at the return signal to detect changes in the environment," Gilmore said. The study was detailed in a recent issue of the journal Science. The ability to produce cytolysin probably evolved as a kind of lethal alarm system to help the bacteria establish islands of safety within their local environments and to keep out unwanted visitors, including other bacteria, scientists say. Over time, the adaptation may have evolved to become a useful weapon, giving the bacteria a means of obtaining nutrients - found within other cells, and released only by rupturing them - that would not otherwise be accessible. The discovery could lead to the development of novel "toxin-inhibitor" drugs that could limit the severity of infections, Gilmore said. Because the bacteria would not be killed directly, there is less chance of them developing a resistance to the drugs. Such a development would be welcome news in hospitals, as more strains of bacteria are becoming increasingly resistant to even the most advanced antibiotics. Source: livescience.com 28 January, 2005 Bacteria Are Genetically Modified by Lightningby Andy Coghlan Lightning is nature’s own genetic engineer. By opening up pores in soil bacteria it allows them to pick up any stray DNA present, report Timothy Vogel, Pascal Simonet and their colleagues at the University of Lyon in France. This hitherto unknown phenomenon might help explain why gene swapping is so common among bacteria. Mild electric shocks are routinely used to genetically engineer bacteria in the lab, so Vogel and Simonet wondered whether lightning could have the same effect. Although it would kill bacteria near the point of contact, those further away would get a milder shock. The researchers persuaded physicist colleagues to blast bacteria with artificial lightning. So far they have shown that two strains of the soil bacterium Pseudomonas - as well as a lab strain of E coli - take up "bait" DNA when zapped by lightning. The researchers suspect the phenomenon is widespread, speeding up the rate at which bacteria evolve. Genetic studies show bacteria frequently pick up foreign genes, usually from other bacteria, but natural DNA uptake rates are too sluggish to explain the observed diversity. Lightning might also have speeded up the evolution of the first bacteria, Vogel says. Source: newscientist.com 19 October 2004 Journal reference: Applied and Environmental Microbiology (DOI: 10.1128/AEM.70.10.6342-6346.2004) S(ub)limeBozeman, Montana - Bacteria are adept at surviving feasts and famines, adjusting their needs and personalities accordingly. Biologists are starting to put these transformations to good use
This is the form in which bacteria prefer to live, but it is not how most people, or even most microbiologists, think of them. Familiar bugs - the ones that cause infectious diseases such as cholera, tuberculosis or tetanus - exist in a more lonely and restless "planktonic" form. In this state, they grow easily in the laboratory, and so they have been much studied. However, for many bacteria, the planktonic way of life is just a brief interlude between two little-known alter egos, the biofilm and something called the "UMB", or ultra-micro bacterium. When food is truly plentiful, bacteria adopt the sedentary life of a biofilm. They clamp themselves to an inviting surface - be it a rock, an artificial joint or a piece of oil-pumping machinery - and switch on the genes that make the thick, slimy covering that gives them their name. This slime both protects them (a bacterium residing in a biofilm is hidden from predators and is 500 times more resistant to antibiotics than it is when free-floating) and acts as a net to catch food. Although biofilms were first recognised in 1978, they have had to wait for almost 20 years for biologists to take them seriously. They are now receiving the attention they deserve, with the opening on 30 September (1996) of the American Society for Microbiology's first conference on biofilms. The renewed interest stems, in part, from a recognition of the havoc that biofilms can wreak. They are implicated, for instance, in some prostate diseases, and in the rejection of artificial joints. But the attention also stems from the discovery that they can be useful. Just Add FoodTo take full advantage of biofilms requires an understanding of the UMB'S biology. If biofilms are what happens to regular bacteria that fall on good times, shrinkage to UMB is what happens when times get bad. In the late 197OS, Richard Morita, a biologist at Oregon State University, in Corvallis, discovered that regular bacteria faced with starvation pack their DNA into tight bundles, shut down virtually all their activities, and shrink down to about 1/3 of their normal size. They can remain in this dormant state for decades, or possibly even centuries, floating around in the deep oceans or buried far underground. (For fun, Dr Morita wakes up one of his favourite specimens once a year. It hasn't failed him yet.) They are hard to detect - UMB forms of cholera cannot be detected in water using standard tests - and once detected, they are too small to observe, except with sophisticated scanning-laser microscopes. When these bacteria eventually encounter a new source of food, they unpack their DNA, switch their genes back on, and resume their former planktonic lives. (The best way to test water for the presence of cholera is to add a lot of food, wait for four hours, and then look for the planktonic form of the critter.) Feed them still more, and they will fatten up and form a biofilm. It is this ability to change from one form to another that makes biofilms potentially useful. Bill Costerton, director of the Centre for Biofilm Engineering at Montana State University, in Bozeman, reckons that changing ultra-micro bacteria into biofilms can provide a way to solve many apparently intractable industrial problems quickly and cheaply. One example comes from the oil industry, which is eager to extract more from its wells. When a well is first drilled, about 15-30% of the oil deposit will typically shoot to the surface simply because it is under pressure. Pumping water into the well, to increase the pressure further, can sometimes recover a further 15-30% of the deposit. But extracting more than that is difficult, for two reasons. "Thief zones" on the deposit's periphery soon begin stealing the injected water, and if the surrounding rocks are porous, capillary pressure keeps oil stuck in the pores. Dr Costerton and his colleagues believe that bacteria can solve both problems. For it turns out that oil wells house a surprising variety of bacterial life. In the early 1990s, the American Department of Energy sampled for microbes while drilling for oil 2,440 metres (8,000 feet) below the earth's surface. At the time, few believed that much microbial life existed so deep underground. But after rigorous testing to rule out contamination, researchers revealed that they had found bacteria - even though the site had experienced no fresh organic input for millennia. his testifies to the longevity of ultra-micro bacteria. But it also suggests a way to plug leaky wells. All bacteria, when starved, take the ultra-micro form. About 60% of them do it quickly and tidily, turning themselves into tiny smooth spheres (the remaining 40% have knobbly bits, not a useful feature from Dr Costerton's perspective). When faced with a leaky well, Dr Costerton samples the bacterial fauna, looking for species that will form smooth shapes. He breeds these in a test tube in their planktonic form, until they reach a concentration of 50 billion per millilitre. At that point he cruelly takes away their food. They continue to grow for a little while, reaching a final concentration of 500 billion per millilitre. But they then enter suspended animation, and Dr Costerton ships them off to their oil well of origin as an unrefrigerated paste. On arrival, they are diluted and injected deep into the well. To turn them into the biofilm that, Dr Costerton hopes, will block up all the holes and prevent leakage, he just adds a chaser of organic waste from distilleries. Presented with such a deliciously rich food, the bacteria unpack themselves, rush through the planktonic phase, and begin life as a film. Chase them with a detergent instead, and they will disrupt capillary pressure in any pores. Not everyone believes that it is necessary to be so elaborate. Alan Sheehy, a biologist working on oil fields in Australia, just adds nutrients to his oil wells, assuming that any bacteria that are down there will readily form a slime if provided with enough food. But Dr Costerton prefers to know the creatures he is dealing with. Moreover, he thinks that his approach can be applied more generally, perhaps to contain polluted sites or to make harmful substances safe. At the moment, he is testing a project to use uMB-turned-biofilm to modify chromium dumps in Scotland. The dumps contain a form of chromium known as chromium(VI). This is an unstable version of the metal, lacking six of the electrons usually present; it is toxic and carcinogenic. The more stable form, chromium(III), is better to have around. One species of bacteria in Dr Costerton's collection naturally converts chromium(VI) to chromium(lII) as it eats. Sprinkle it on the ground in its ultra-micro state, and it will sink 200 - 300m into the earth (in its planktonic form, it would get stuck after about lcm). Adding food then activates the bacteria, allowing them to get to work converting the metal. Not all biofilms are so handy. The UMB usually hang out harmlessly in the bowels of the earth and the oceans. But biofilms are everywhere: on teeth, inside the gut and the bladder, on rocks, roofs and machines. Although they rarely cause disease, they are hard to get rid of when they do. Luckily, they are conformists; one biofilm behaves much like another. As more is learnt about them, they should become more helpful and less hazardous - perhaps even to hapless hikers. Source: The Economist 5 October 1996 There are several article in this section dealing with unicellular life, including these:
For articles on bacteria, centrioles, chairs, nebulae, asteroids, robots, memory, chirality, pain, fractals, DNA, geology, strange facts, extra dimensions, spare parts,
discoveries, ageing and more click the "Up" button below to take you to the Table of Contents for this Science section. |
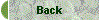
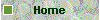
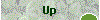
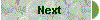