Redshifts and Pentaquarks
A Very Different UniverseA universe that came from nothing in the big bang will disappear into nothing at the big crunch. - Paul Davies The universe is a big place, perhaps the biggest. - Kurt Vonnegut Cosmologist Edward Harrison With the enormous successes of Big Bang cosmology and inflationary-universe theory, the "standard model" of the universe goes almost unquestioned by cosmologists these days. In this picture the redshifts of distant galaxies accurately indicate the expansion of space, the Big Bang happened around 10 or 15 billion years ago, and, very early in the Big Bang, inflation gave the cosmos precisely the right density to balance it forever between rapid expansion and re-collapse. That's where many lines of observation and theory all seem inexorably to point. Or maybe not. As an exercise, cosmologist Edward Harrison (University of Massachusetts, Amherst) tried to devise a radically different universe that passes all the major observational tests. He succeeded surprisingly well. In Harrison's most extreme model of the cosmos, the expansion is very slow, with a Rubble parameter of only 10 kilometers per second per megaparsec (1 megaparsec is 3.26 million light-years). This means the density of the universe today is about 10 times greater than needed to halt the expansion. In this scenario astronomers are misled to think the Rubble parameter is 50 to 100 because the high density promotes the formation of very large, massive structures in the extreme distance. Their gravity is pulling galaxies and quasars away from us in all directions, causing the observed redshifts. In Harrison's model, redshifts don't tell anything at all about cosmic expansion until you look more than a billion light-years away. This is beyond the range of most other methods for measuring galaxy distances that astronomers currently use. How old is a high-redshift galaxy or quasar? The "standard model" of the Harrison's universe is 35 billion years old. This provides ample time to accommodate old stars and globular clusters that appear to have ages of around 17 billion years - a troublesome squeeze to fit into the standard model. The universe will cease expanding in another 22 billion years, then begin recollapsing toward a Big Crunch 79 billion years in the future. This universe is limited in size as well as in time. Right now it's only 100 billion light-years to the antipode, the opposite side of our "closed cosmic hypersphere." We should be able to see objects that are almost that far right now (they were much nearer when their light left them). By contrast, inflation theory makes the universe essentially flat and fantastically larger than can be observed, by dozens or perhaps millions of orders of magnitude. Harrison's model has its advantages. The problem of what could have started the largest structures forming pretty much goes away. So does the need for exotic, unknown types of dark matter. As for the violation of inflation's dictum that the cosmic density will perfectly balance the expansion, Harrison points out that we don't really know what happened during or right after inflation (assuming it happened at all) and shouldn't be too glib about it. He is especially bothered by cosmologists assuming that redshifts can tell them the true expansion of space unless they choose a density value first. The success of his low-expansion, high-density universe reminds us, Harrison says, "of the need to be more agnostic in our attitude toward current cosmological beliefs." His paper appears in the March 1st Astrophysical Journal Letters. Source: Sky & Telescope September 1993 Will It Ever End?by Tim Radford Several masters of the universe are in mortal combat over the birth and survival of time itself. Two papers circulating among mathematicians and physicists are wrestling with the problem of why the universe might never end. On the one hand, the wheelchair-bound cosmologist Stephen Hawking – probably the world's most famous living scientist – and his Cambridge University colleague Neil Turok, argue in a paper published in the journal Physics Letters that what happened in the first trillion-trillion-trillionth of a second of time may dictate an eternity of desolation for the universe. On the other hand, Russian physicist Andrei Linde, one of the giants of inflation theory (which tries to explain what happened inside that first small fraction of a second) has circulated a paper saying Hawking and Turok have got it wrong: universes like ours are popping into existence all the time, so there's no point in trying to find a beginning, or an end. At the bottom of the argument is a big problem. All the evidence says the universe has a beginning, and that includes space and time. The universe has been expanding for about 15 billion years. So was there a "before" this moment of creation? And will the expansion end? Ten years ago, in the best-selling A Brief History Of Time, Hawking proposed that the universe exploded from a single point in which the force of expansion was forever being slowed by the gravitational power of the matter in it. Which would win? Would the universe expand forever, or would it collapse back on itself in a universal black hole? In the past few months, astronomers have repeatedly proposed that the universe is not dense enough to stop its own expansion. After billions of years, all the galaxies will have faded, but their cinders will go on sailing away from each other for eternity. Hawking’s latest paper examines some of Einstein's thinking – and uses pure theory to reach the same conclusion: the future of the universe was decided by the conditions in that first moment of its making. Professor Sir Martin Rees, Britain's Astronomer-Royal said: "They claim they get a cosmology with a low density in a more natural way than other ideas along these lines. This is a variant that makes use of some of Steve's earlier ideas." He added that Linde claims the Hawking and Turok model does not give the right density for the universe. "Their idea has been criticised by the high priest already." Both arguments depend on a moment called "cosmic inflation," in which a universe popped up from literally nowhere and expanded far faster than the speed of light, inflating itself. This inflation was a kind of anti-gravity. But, the argument says, since gravity is negative energy, then this anti-gravity must have represented positive energy. Einstein's theories say matter is just frozen energy, so all the stars and galaxies are condensed from the energy manufactured from nothing in this inflationary moment. Inflation has given astronomers 17 years of fun. Does it leave a universe that is critically balanced between expansion and collapse, or one with "negative curvature" and an open-ended future? "That's what Linde and Hawking and Turok are trying to understand," said Sir Martin. "They are trying to get a variant of inflation that allows you to end up with a universe that is uniform but has negative curvature. The new thing in the Hawking-Turok paper is to show you could do this rather more naturally." The new Hawking argument means philosophers now have to think about time having a beginning but no end. It could be worse. "Linde believes in what he calls eternal inflation," said Sir Martin. "Once you set a universe going, it inflates and sprouts new big bangs all the time. One of Linde's criticisms is that Hawking talks about the initial big bang, but there never really was an initial big bang. Once you set up one, it sprouts an infinite number of big bangs. If that is the case, then the initial conditions Steve worries about are lost in even deeper mists of cosmic history than we expected." Source: The Guardian 24 February 1998 See also:
The Universe Is Flat, Say CosmologistsThe universe, they said, depended for its operation on the balance of four forces - Terry Pratchett The usual approach of science of constructing a mathematical model cannot answer - Stephen W Hawking by David Derbyshire More than 500 years after Columbus proved that the Earth was round, cosmologists have shown that the universe is flat. The most detailed study of its kind has revealed that space is not curved, that light usually travels in straight lines and that parallel lines will only meet at infinity. The finding, heralded as a major advance in astronomy, also rules out a cataclysmic "big crunch" in which the universe will eventually implode. The shape of the universe has been one of the great questions of cosmology. If it is flat, then the rules of Euclidean geometry taught at school apply to most of the universe. But if it is curved, as many astronomers have argued, then straight lines are really curved and light travels in arcs. Clues to the shape of the universe come from ripples in the microwave radiation left behind 300,000 years after the Big Bang. These records show the structure of the universe when it was 1,000 times smaller and hotter than it is today. A map of the infant universe was created by researchers working on Boomerang - the Balloon Observations of Millemetric Extragalactic Radiation and Geophysics - who report their conclusions in Nature. The project used a telescope suspended from a balloon to circumnavigate the Antarctic at 3650 metres for 11 days in 1998. The telescope recorded about one billion faint signals. An analysis of the signals and subtle differences in temperature showed the geometry of the infant universe. It also supported the inflationary theory which states that the universe expanded by an incredible amount in the split second after the Big Bang 12 to 15 billion years ago. That expansion stretched the universe in all directions to such a large extent that it became effectively flat. The shape of the universe depends on its mass. Cosmologists have come up with three possible shapes. One is curved and open like a bowl, another is curved and closed like a sphere and the third is flat. Above a certain critical mass, the universe must be closed. Eventually it will stop expanding and collapse back on itself. A two-dimensional analogy is a sphere. Below a critical mass, the universe has to be open and will keep on expanding forever. An open universe can be represented by an open bowl. But if the universe is perfectly balanced between these two, it is described as flat. It will continue to expand, but at a slower rate. Though the Euclidean geometry applies to most of the universe, there ate regions where space is curved by a strong gravitational field, for instance around a black hole. Astronomers cannot account for all the matter or energy needed to make the universe flat. To cope with the massive discrepancy, they have proposed the existence of mysterious dark matter and dark energy. The latter would be almost like antigravity, and would push space apart. - Daily Telegraph Source: The Dominion (Wellington, NZ) 3 May 2000 Phantom Energy and Cosmic Doomsdayby Robert R Caldwell, Marc Kamionkowski, Nevin N Weinberg Cosmologists have long wondered whether the universe will eventually re-collapse and end with a Big Crunch, or expand forever, becoming increasingly cold and empty. Recent evidence for a flat universe, possibly with a cosmological constant or some other sort of negative-pressure dark energy, has suggested that our fate is the latter. However, the data may actually be pointing toward an astonishingly different cosmic end game. In our abstract we explore the consequences that follow if the dark energy is phantom energy, in which the sum of the pressure and energy density is negative. The positive phantom-energy density becomes infinite in finite time, overcoming all other forms of matter, such that the gravitational repulsion rapidly brings our brief epoch of cosmic structure to a close. The phantom energy rips apart the Milky Way, solar system, earth, and ultimately the molecules, atoms, nuclei, and nucleons of which we are composed, before the death of the universe in a "Big Rip." Robert R Caldwell can be contacted at robert.r.caldwell@dartmouth.edu Source: arxiv.org Tuesday 25 February 2003 See also:
Physics Team Goes Where No Quark Has Gone BeforeMagnetism is one of the Six Fundamental Forces of the Universe, with the other five being Gravity, Duct Tape, Whining, Remote Control, and The Force That Pulls Dogs Toward The Groins Of Strangers. - Dave Barry Physicists have discovered a new class of subatomic particles, offering unexpected insights into the building blocks of matter. The discovery involves tiny particles called "quarks," the bricks and mortar of protons and neutrons in the atomic nucleus. Until now, physicists had only seen quarks packed into two- or three-quark combinations inside the larger subatomic particles. These combinations have always been something of a mystery. In their efforts to unravel the secrets of matter, scientists have tried for three decades to come up with different combinations. And now a Japanese team led by Takashi Nakano of Osaka University says it has created a five-quark particle - "pentaquark" - in an experiment at the SPring-8 physics lab. Testing a theory from Russian scientists, the team blasted carbon atoms with high-energy X-rays to make the pentaquarks. Determining why the pentaquark appeared in the experiment should offer great insight into the nature and stability of the essential building blocks of all matter, says physicist Ken Hicks of Ohio University in Athens, who took part in both the experiment and a confirmatory effort at the US Department of Energy's Thomas Jefferson National Accelerator Facility. "It took me two months to convince myself this was real," Hicks says. "It has been a real roller coaster." Quarks come in six types, or "colours." The type of quarks inside protons and neutrons determines the mass, energy and magnetism of those particles. The pentaquark's stability likely comes from a unique combination of quarks, says physicist Peter Barnes of Los Alamos (NM) National Lab. The findings will appear in Friday's Physical Review Letters. Source: USA Today 1 July 2003 Little Albert and big Godby Bryan Appleyard Last year universities around the country closed physics departments. The subject was widely undersubscribed and, in the eyes of our wonderful modernising government, it didn’t actually seem to do anything useful. One less physics department was about as bad for the economy as one less theology faculty. Both theologians and physicists seem to pass their days in futile speculation about the meaning of everything without ever saying anything useful or comprehensible. They may as well do that on their own time, spending their own money. This year is Einstein Year. It will be the 100th anniversary of the “miracle year” in which Albert Einstein, at the age of 26, overturned almost every orthodoxy of post-Newtonian physics. He did it on his own time, spending his own money. He was a patents clerk in Berne in Switzerland: no university would have him. It will also be the 50th anniversary of his death. He departed this life in April 1955 muttering on a hospital bed in Princeton, New Jersey. What he murmured we do not know. The nurse attending him spoke no German and, at the last, he had returned to his native tongue. By his bed were pages of equations which went nowhere and meant nothing. Or did they? The physics lobby — quite rightly, of course — is seizing on 2005 as a chance to fight back against the reduction of the subject. There will be events around the country, and the Institute of Physics has even adopted a rap song by DJ John Vader — Einstein (Not Enough Time) — as a kind of theme tune. “Listen to the Vader,” it goes. “Size, passing through like warp factor nine / Delivering the message out there from the legend Einstein.” Judging by the website (einsteinyear.org) the celebrations will be a combination of the usual fun, with the underlying message that Einstein’s work was of practical importance. This is true enough. Aircraft might be landing up to 15 miles from Heathrow, and your car’s sat nav would never get you home if GPS (global positioning system) technology had failed to factor in relativity. Einstein was also one of the first formulators of quantum theory, without which we would have no computers. On the whole, however, the feeling is that Einstein said some amazing things about the universe which had little or no impact on the practicalities of our daily lives. Understandable though this may be, it is a feeling that physicists are most anxious to refute. It is a secular impulse for a secular society and it is, frankly, boring. More to the point, it is philistine. The real reasons Einstein still inspires and thrills — and will do so for as long as this quantum, relative space deigns to support human life — are, above all, religious and aesthetic. Speculative physicists are driven people. All too frequently they are driven to say staggeringly stupid things when they step outside their own discipline. But the reason they are driven is entirely noble and dignified. It is the same reason that inspires prophets and great artists. They think they are on to something fundamental without quite knowing what. Next to that, all else pales into insignificance. Einstein’s struggle was with God and only God. To understand this it is necessary to understand at least the style of his thought. He was a very specific kind of thinker. As a mathematician he was quite weak. Most great physicists are also great mathematicians and, as a result, tend to see problems from the bottom up: working from the numbers upwards to the big theory. Einstein, however, was a top-down thinker. He started from the top and worked downwards to the details. It was his greatest strength but also his greatest weakness. He hit upon special relativity, the biggest scientific achievement of 1905, because he asked a typical top-down question: what would it be like to sit on a beam of light? This led to the revelation that the speed of light was the one constant in the universe. Everything else (time included) was relative to the position and speed of the observer. In 1915, with his theory of general relativity, Einstein extended this hypothesis to include gravitation. Gravity was the result of the curvature of space time in the vicinity of large bodies. Gravity also travelled at the speed of light (Newton thought this action was instantaneous). A good, smart party trick is to summarise the theory of relativity in less than 10 words. It can be done in seven: action at a distance happens in time. From 1915 onwards Einstein was a global star: the face of modern genius at a time when it really did seem we were on the brink of a final vision of cosmic truth. In 1929 a new paper of his was displayed in the windows of Selfridges department store. It was a sacred object — a message from the god of physics and maybe the maker of all things. The equivalent in our time was, it could be argued, the appearance of Stephen Hawking on Star Trek and The Simpsons. But that particular 1929 paper was trashed by Einstein’s peers, as was almost everything else he wrote after 1915. The reason was that his pursuit of God seemed to have led him astray. At the heart of everything Einstein thought in his top-down way was the conviction that the universe was humanly comprehensible as well as being beautiful and — in essence — simple. That, he believed, was how God would have made it. The problem was that Einstein’s own science seemed to lead to a denial of any such possibility. First, there was the problem of why the universe was not apparently contracting into a conclusive Big Crunch under the force of gravity. He solved this by inventing the “cosmological constant”, a repulsive force that would balance the attractive force of gravity. But this was both mathematically ugly and, in terms of science at the time, unsustainable. Einstein subsequently dismissed the cosmological constant as his greatest blunder. Second, quantum theory had become the bizarre world of quantum mechanics in which causality collapses and classical physics finds itself confronted with unscaleable barriers. Quantum effects are also inconsistent with relativity effects, an inconsistency we are still a long way from resolving. Einstein believed, in opposition to leading physicists of the era, that this was only an apparent effect caused by the fact that we did not know enough. In reality, there were "hidden variables", which would restore the quantum flux to the bosom of classical physics. Right up to the moment of his death Einstein struggled to produce his own theory of everything, on the basis of scientific notions that everybody else was convinced were utterly wrong. In the 1930s the universe had been shown to be expanding, so the cosmological constant seemed to be erroneous. At the same time the explanatory power of quantum mechanics was overwhelming. Einstein was on his own — locked into stardom but out of science. The irony now is that Einstein may have been partly right. In its exotic way, physics has returned to the possibility of the cosmological constant. String theory, M theory and even the quaintly named “quintessence theory” all suggest there must be a repulsive force to counteract gravity. "The cosmological constant that Einstein considered, discarded, reconsidered, and then abandoned, must be reconsidered again," says physicist Paul Davies in a soon-to-be published article. Quantum mechanics, however, still remains far beyond the reach of Einstein’s classicising imagination. Nevertheless, in the late 1980s and early 1990s, physicists such as Hawking in Britain and Steven Weinberg in America were promising a theory of everything based on the unification of quantum theory and relativity. Einstein, it seemed, was wrong to believe that the irrational quantum chaos at the heart of everything would prevent any such theory. But Hawking and Weinberg were wrong. The theory of everything is as far away today as it ever was. It may be that it can never happen. Einstein could have been right again: quantum uncertainty may make such a theory impossible or the fact that we know arithmetic to be uncompletable may mean we can never achieve such a synthesis. Nonetheless, the search goes on, driven by people convinced they are on to something without quite knowing what. Difficult as Einstein’s science might appear to be, there is nothing complicated or obscure about the issue it raised and tried to address, for it is the same issue we all daily raise and try to address — the nature of the world into which we have been flung. Is it formed on the basis of a scheme accessible to human reason — a pattern both beautiful and simple. A pattern worthy, perhaps, of God? Einstein thought so. Or is it chaotic, beyond all possibility of human rational analysis? Many now think that it must be so. When the toast falls butter-side down is something or somebody to blame, or is it just life? There is no final evidence either way. Rather, there is no evidence at all, only straws in a wind which keeps changing direction. Which position you take is therefore a matter of subjective inclination. It may even vary from day to day. It will, nevertheless, condition everything you do and are. All of which is to say that physics is as important as theology, and our fantasies of secularity will never stop our pursuit of both. So, this year remember great Albert, physicist, artist and theologian, and his last mutter which, I have always secretly hoped, was: “Ah, I see!” He had earned that much. Source: timesonline.co.uk The Sunday Times - Review 2 January 2005 Process Physics
A new paradigm for the modelling of reality is currently being developed called Process Physics. Process Physics starts from the premise that the limits to logic, which are implied by Gödel's incompleteness theorems, mean that any attempt to model reality via a formal system is doomed to failure. Instead of formal systems, a process system is preferred which uses the notions of self-referential information with self-referential noise and self-organised criticality to create a new type of information-theoretic system that realises both the current formal physical modelling of reality but also exhibits features such as the direction of time, the present moment effect and quantum state entanglement (including EPR effects, nonlocality and contextuality), as well as the more familiar formalisms of Relativity and Quantum Mechanics. In particular a theory of Gravity has already emerged. In short, rather than the static 4-dimensional modelling of present day (non-process) physics, Process Physics is providing a dynamic model where space and matter are seen to emerge from a fundamentally random but self-organising system. The key insight is that to adequately model reality one must move on from the traditional non-process syntactical information modelling to a process semantic information modelling; such information is "internally meaningful." The new theory of gravity which has emerged from Process Physics is in agreement with all experiments and observations. This theory has two gravitational constants: G, the Newtonian gravitational constant, and a second dimensionless constant which experiment has revealed to be the fine structure constant. This theory explains the so-called "dark matter" effect in spiral galaxies, the bore hole gravitational anomalies, the masses of the observed black holes at the centres of globular clusters, and the anomalies in Cavendish laboratory measurements of G. The experimental and theoretical research program to study and develop this theory of gravity is supported by an Australian Research Council Discovery Grant. Source: scieng.flinders.edu.au Lab Fireball "May Be Black Hole"Creating the conditions for the formation of black holes is one of the aims of particle physics A fireball created in a US particle accelerator has the characteristics of a black hole, a physicist has said. It was generated at the Relativistic Heavy Ion Collider (RHIC) in New York, which smashes beams of gold nuclei together at near light speeds. Horatiu Nastase says his calculations show that the core of the fireball has a striking similarity to a black hole. His work has been published on the pre-print website arxiv.org and is reported in New Scientist magazine. When the gold nuclei smash into each other they are broken down into particles called quarks and gluons. These form a ball of plasma about 300 times hotter than the surface of the sun. This fireball, which lasts just 10 million, billion, billionths of a second, can be detected because it absorbs jets of particles produced by the beam collisions. But Nastase, of Brown University in Providence, Rhode Island, says there is something unusual about it. Ten times as many jets were being absorbed by the fireball as were predicted by calculations. The Brown researcher thinks the particles are disappearing into the fireball's core and reappearing as thermal radiation, just as matter is thought to fall into a black hole and come out as "Hawking" radiation. However, even if the ball of plasma is a black hole, it is not thought to pose a threat. At these energies and distances, gravity is not the dominant force in a black hole. The RHIC is sited at the Brookhaven National Laboratory. Source: news.bbc.co.uk BBC News 17 March 2005 © BBC MMV NASA Achieves Breakthrough In Black Hole Simulation
Click to play Source: Henze, NASA NASA scientists have reached a breakthrough in computer modeling that allows them to simulate what gravitational waves from merging black holes look like. The 3-dimensional simulations, the largest astrophysical calculations ever performed on a NASA supercomputer, provide the foundation to explore the universe in an entirely new way. According to Einstein's math, when two massive black holes merge, all of space jiggles like a bowl of Jell-O as gravitational waves race out from the collision at light speed. Previous simulations had been plagued by computer crashes. The necessary equations, based on Einstein's theory of general relativity, were far too complex. But scientists at NASA's Goddard Space Flight Center in Greenbelt, Maryland have found a method to translate Einstein's math in a way that computers can understand. A New ModelThis visualization shows what Einstein envisioned. Researchers crunched Einstein's theory of general relativity on the Columbia supercomputer at the NASA Ames Research Center to create a 3-dimensional simulation of merging black holes. This was the largest astrophysical calculation ever performed on a NASA supercomputer. The simulation provides the foundation to explore the universe in an entirely new way, through the detection of gravitational waves. "These mergers are by far the most powerful events occurring in the universe, with each one generating more energy than all of the stars in the universe combined. Now we have realistic simulations to guide gravitational wave detectors coming online," said Joan Centrella, head of the Gravitational Astrophysics Laboratory at Goddard. The simulations were performed on the Columbia supercomputer at NASA's Ames Research Center near Mountain View, California. This work appears in the 26 March issue of Physical Review Letters and will appear in an upcoming issue of Physical Review D. The lead author is John Baker of Goddard. Similar to ripples on a pond, gravitational waves are ripples in space and time, a 4-dimensional concept that Einstein called spacetime. They haven't yet been directly detected. Gravitational waves hardly interact with matter and thus can penetrate the dust and gas that blocks our view of black holes and other objects. They offer a new window to explore the universe and provide a precise test for Einstein's theory of general relativity. The National Science Foundation's ground-based Laser Interferometer Gravitational-Wave Observatory and the proposed Laser Interferometer Space Antenna, a joint NASA - European Space Agency project, hope to detect these subtle waves, which would alter the shape of a human from head to toe by far less than the width of an atom. Black hole mergers produce copious gravitational waves, sometimes for years, as the black holes approach each other and collide. Black holes are regions where gravity is so extreme that nothing, not even light, can escape their pull. They alter spacetime. Therein lies the difficulty in creating black hole models: space and time shift, density becomes infinite and time can come to a standstill. Such variables cause computer simulations to crash. These massive, colliding objects produce gravitational waves of differing wavelengths and strengths, depending on the masses involved. The Goddard team has perfected the simulation of merging, equal-mass, non-spinning black holes starting at various positions corresponding to the last two to five orbits before their merger. With each simulation run, regardless of the starting point, the black holes orbited stably and produced identical waveforms during the collision and its aftermath. This unprecedented combination of stability and reproducibility assured the scientists that the simulations were true to Einstein's equations. The team has since moved on to simulating mergers of non-equal-mass black holes. Einstein's theory of general relativity employs a type of mathematics called tensor calculus, which cannot be turned into computer instructions easily. The equations need to be translated, which greatly expands them. The simplest tensor calculus equations require thousands of lines of computer code. The expansions, called formulations, can be written in many ways. Through mathematical intuition, the Goddard team found the appropriate formulations that led to suitable simulations. Progress also has been made independently by several groups, including researchers at the Center for Gravitational Wave Astronomy at the University of Texas, Brownsville, which is supported by the NASA Minority University Research and Education Program. Source: nasa.gov 18 April 2006 For articles on bacteria, centrioles, chairs, nebulae, asteroids, robots, memory, chirality, pain, fractals, DNA, geology, strange facts, extra dimensions, spare parts, discoveries, ageing and more
click the "Up" button below to take you to the Index for this Science section. |
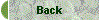
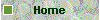
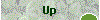
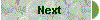